ABOVE: The response of pigs to pathogens is complicated in commercial settings through the use of antimicrobial growth promoters and vaccination protocols. Photo: Barbara Barbosa
Continuing on from the October issue, Part 2 of this review will address the immune challenges in pork production.
Stimulation of the immune system results in higher concentration of circulating acute-phase proteins, immune cell proliferation, secretion of various molecules secreted by immune cells and lymphoid tissue hyperplasia, with AA necessary to support the activated immune system.
Though the amount of AA required for the activated immune system is not a substantial component of whole-body protein turnover, the AA profile required to support the immune response is drastically different than for muscle protein synthesis, resulting in a disparity between AA requirement and supply and reduction in utilisation efficiency.
A significant expenditure of AA during inflammation is associated with the syntheses of endogenous antioxidants to cope with oxidative stress.
Though the mitochondrial electron transport chain is a major site of free radical production, immune cells, such as phagocytes and monocytes, produce free radicals in the form of bactericidal agents or during the process of producing immune-signalling compounds.
Accumulating evidence has shown that dietary supplementation of specific AA can strengthen the pig’s immune system by minimising body protein loss and accelerating its recovery.
This strategy seems to be more relevant for nursery pigs, who commonly undergo ISS post-weaning period.
Enteric pathogen challenge
The most common enteric diseases at pig farms are salmonellosis and swine enteric colibacillosis characterised by neonatal diarrhea and post-weaning diarrhea.
In-feed antimicrobial growth promoters have been widely used to prevent or suppress the effects of enteric diseases, however due to the ban on their use in many jurisdictions, animal nutritionists have made great efforts to seek alternative means to enhance gastrointestinal health while reducing antibiotic use.
Though enteric pathogens have different pathotypes and pathogenicity, they all infect intestinal epithelial cells, triggering the action of the host’s immune system, generally leading to diarrhea.
These toxins activate the cyclic guanosine monophosphate and cyclic adenosine monophosphate systems, resulting in disruption of chloride and ion channels and, eventually, osmotic diarrhea.
Similarly, ST attaches to the intestinal epithelial cells and delivers a specialised set of effectors.
Subsequently, ST injects salmonella invasion protein into the intestinal epithelial cells, leading to cytoskeletal rearrangement in host cells, disruption of the normal epithelial brush border and activation of the immune system.
The infected cells lose electrolyte absorption capabilities, thereby leading to diarrhea.
Intestinal inflammation and disrupted integrity are common observations in pigs challenged with ETEC or ST.
Inflammation and redox signalling systemically govern the fate and permeability of intestinal epithelial cells, which are relevant to intestinal integrity.
Pro-inflammatory cytokines trigger mitogen-activated protein kinase signalling and lead to apoptosis of enterocytes on the villus, mainly at the tip.
Furthermore, the expression of myosin light-chain kinase, which causes cytoskeletal contraction, is activated under inflammatory conditions, thereby decreasing intestinal permeability.
Pigs challenged with ETEC or ST are characterised by poor intestinal morphology (for example villus height, villus width and crypt depth) and permeability, as well as reduced brush border enzyme activity and nutrient transporter expression compared to non-challenged counterparts.
As enteric pathogens eventually stimulate the systemic immune system, the metabolic pathways change.
These changes are initiated by the immediate increase in concentration of acute-phase proteins and immunoglobulins following pathogen exposure.
As a result, the requirements for several AA, such as Thr, Trp, and sulfur AA, may be elevated for optimal growth performance and immune response.
Respiratory pathogen challenge
As a result of intensive confined systems of modern pig production, respiratory diseases continue to be a cause of concern in pork production, commonly resulting in impaired weight gain and feed efficiency, poor animal welfare and increase in medication costs.
There are several reports from different parts of the world indicating significant prevalence of pneumonia (20 to 80 percent) and pleuritis (4 to 60 percent) in slaughtered pigs, which indicates an important impairment of the respiratory tract throughout the productive life and potentially significant productive costs of immune stimulation.
The term porcine respiratory disease complex is often used to address the several bacteria, viruses and mycoplasmas involved in the etiology of respiratory diseases.
The most important agents are actinobacillus pleuropneumoniae, mycoplasma hyopneumoniae, pasteurella multocida, porcine circovirus type 2, porcine reproductive and respiratory syndrome virus, porcine respiratory coronavirus and salmonella choleraesuis.
The growth performance response of pigs to respiratory pathogens will be dependent on the agent involved.
In general, respiratory diseases were less detrimental to performance than the other immune challenges, which may possibly be due to a greater degree of compensatory growth following respiratory infections.
Moreover, many respiratory pathogens produce an immune response that is more likely to be contained within the affected tissues, resulting in more minor clinical response and a reduction in a significant response in growth performance.
However, subclinical infections can still result in significant economic consequences.
Among respiratory pathogens, there is more evident information in the literature to show that PRRSV is the most detrimental to performance.
PRRSV infection reduces growth performance through a combination of reduced feed intake and decreased nutrient utilisation.
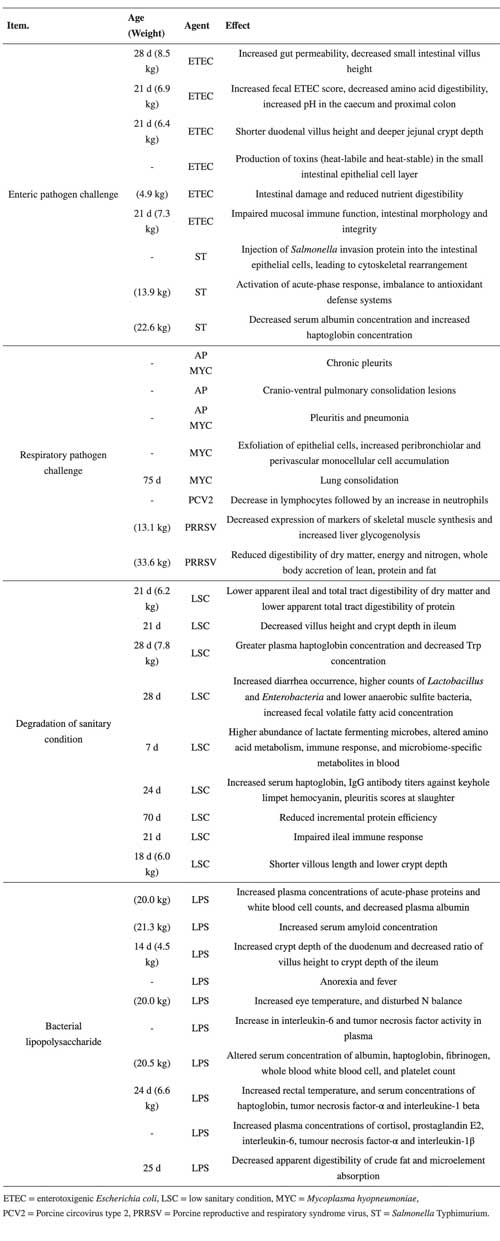
Table 1: Mechanisms through which challenge models impact nutrient digestion and metabolism and growth performance.
Degradation of sanitary condition
While it is important to understand the effects of individual pathogens on pig immune status and performance, in commercial settings pigs are more likely to be exposed to a variety of pathogens.
This exposure is the result of an increase in environmental pathogen load.
Moreover, the response of pigs to pathogens is complicated in commercial settings through the use of antimicrobial growth promoters and vaccination protocols, which reduce pathogen load and enhance herd immunity to pathogens and individual pig ability to avoid infection.
Experimentally, sanitary challenge represents an increase in pathogen exposure via absence of cleaning and disinfection protocols, reduction in vaccination, reduction in antibiotic use or a combination.
Pigs are exposed to various stressors, broadly classified as non-biological stressors and biological stressors.
The sanitation challenge aims to mimic the practical commercial swine barn environment and mildly activate the pig’s immune system and is thus often regarded as subclinical compared to studies of other pathogen, toxin and stress challenges.
Nevertheless, this model has been advantageously adopted by studies that tested diet interventions for pigs raised in commercial environments and in which nutrient requirements of pigs housed in experimental or commercial environments were compared.
Because the sanitation challenge model can be applied without special facility or inoculum preparation, the experimental design is more flexible than that of other challenge models.
Due to this design flexibility, the model can be applied to a large number of pigs, which increases the statistical reliability of growth performance.
Because LSC are usually created by the accumulation of manure in the pens, the pigs are more likely to ingest pathogens from the manure orally.
Thus, gut health indicators, such as intestinal integrity and microbial composition, are typically investigated in sanitary challenge studies.
Furthermore, pathogens or dust can be transferred into the body through the nasal tract, so respiratory health indicators are also of interest in these studies.
Indeed, LSC increased the incidence of respiratory tract inflammation, possibly because of the increased loads of respiratory pathogens.
Additionally, LSC are often characterised by the build-up of noxious gases, such as hydrogen sulfide and ammonia, as well as dust, which possibly irritate the respiratory tract.
Pigs raised in LSC generally have lower body weight gain compared to those raised in high sanitary conditions.
However, whether this low body weight gain is accompanied with low feed intake has been argued.
Moreover, it has been consistently reported that intestinal inflammation, oxidative stress and disturbed intestinal integrity are consequences of housing pigs in LSC.
Additionally, shorter villus height in the small intestine have been observed in pigs reared in LSC compared to those reared in HSC.
The change in intestinal integrity may explain the lower ATTD of dry matter, nitrogen and gross energy in pigs housed in LSC.
Moreover, the physiological changes caused by LSC alter nutrient and energy metabolism, leading to shifts in AA and maintenance energy requirements.
A change in the abundance of lactic acid-producing bacteria has been noticeable in sanitary challenge studies.
One such study used metagenomic assay and showed that the megasphaera genus, known to be involved in lactate fermentation, was lower in the colon digesta of pigs reared in LSC.
A reduced number of megasphaera may be associated with lower concentration of lactic acid in the lumen.
A general agreement exists that LSC results in greater protein fermentation.
Recent studies have consistently reported that LSC reduce butyrate concentrations in the colon and faeces.
These findings were consistent with microbial assays in which the abundance of butyrate-producing bacteria, such as clostridiales family XIII incertae sedis and clostridium IV and XIVa, decreased in LSC-challenged pigs.
In contrast to butyrate, sanitary degradation generally increases total short chain fatty acids and volatile fatty acids concentration.
Te Pas et al reported that LSC increases the abundance of lachnospiraceae family in the colon digesta, which are known to be one of the major microbes involved in SCFA synthesis from complex polysaccharides.
Montagne et al also reported an increase in VFA concentrations in the faeces of nursery pigs housed in LSC.
The authors postulated this phenomenon as the ‘hygiene hypothesis’, meaning that a clean environment can impede the optimal development of the immune system and microbiome establishment in young animals.
However, LSC might decrease foregut digestion and increase undigested nutrients, thereby modifying hindgut fermentation patterns.
Therefore, further studies are necessary to elucidate changes in fermentation patterns and microbial metabolites in relation to sanitary conditions.
Though the sanitary challenge model can be applied to nutritional studies, the model has been criticised due to the lack of reproducibility.
While other immune challenge models can quantify the stimulation intensity (for example dose), quantifying sanitation level is difficult because the sanitary challenge model generates various stressors in different facilities and environments.
Swine barns have different predominant pathogens and pathogenesis, causing the intensity of the stressors to vary depending on location.
Furthermore, farms have different floor systems (such as slat and pit), which may affect the stressors’ intensity as this directly influences the quantity of manure accumulation and noxious gas exposure.
These factors lead many studies to report no differences in growth performance or ISS between sanitary conditions.
Therefore, to improve the reproducibility of the sanitation challenge model, details of experimental procedures and environments, including air quality, ventilation operation, ambient temperature, humidity, manure source and management, pen cleaning frequency, pen size and floor design should be provided.
Bacterial lipopolysaccharide
Lipopolysaccharide is a component of the outer membrane of gram-negative bacteria implicated in the pathogenesis of many disease states, causing endothelial cell injury and dysfunction.
The LPS possesses a highly proinflammatory characteristic, which triggers the upregulation of cytokines, adhesion molecules and tissue factor.
The model has been largely used in pigs to mimic systemic inflammation and causes significant reduction in body weight gain.
It should be noted that the period after which LPS is administered may influence the response in growth performance of pigs, since tolerance may occur following multiple injections.
A recent meta-analysis conducted by Rodrigues et al revealed a greater reduction in average daily gain in studies using LPS compared to studies using other challenge models (for example respiratory pathogens, low sanitary condition, environmental stress).
This is consistent with sepsis and septic shock entailed by LPS administration mainly due to the overt cytokine production.
Among them, IL-6 circulating concentration, which is associated with fever, has been reported to increase after LPS injection.
Pastorelli et al reported in a meta-analysis that the reduction in performance during a LPS challenge was due to a depression in feed intake and not to increased maintenance requirements.
More recently, these findings were contradicted by another meta-analytical approach, which statistically contrasted different challenge models and showed that maintenance requirements had a substantial contribution to the decreased performance in LPS-challenged pigs.
Indeed, altered AA requirements have been demonstrated in multiple studies in LPS-challenged pigs, including Met, Thr and Trp, which indicates that nutrient maintenance requirements/utilisation efficiency play an important role in the observed reduction in performance.
Impairment in growth performance has been reported in weaned pigs, along with fever and increased production of major acute-phase proteins and cytokines (such as haptoglobin, tumor necrosis factor-α and interleukine-1 beta).
This is consistent with the findings of Xu et al, who reported an increased plasma concentration of cortisol, prostaglandin E2, IL-6, TNF-α and IL-1β dramatically after LPS administration, which corroborates the systemic commitment after challenge.
A recent study revealed the clear reduction in nutrient utilisation efficiency in LPS-administered pigs, where not only growth performance but also the concentration of most microelements in faeces and the expression of most microelement transport genes in the mucosa of the gastrointestinal tract were decreased after injection.
It is important to consider that LPS is a challenge model mainly used to mimic systemic activation of the immune system.
However, since many pathogens and antigens are able to entail systemic response, it may be inferred that nutrient requirements are increased when there is a load to the production of immune cells and co-factors.
Part 3 in next month’s issue will feature nutritional strategies for improved health status.
Note that the article in its entirety can be viewed at ncbi.nlm.nih.gov/pmc/articles/PMC9598274/